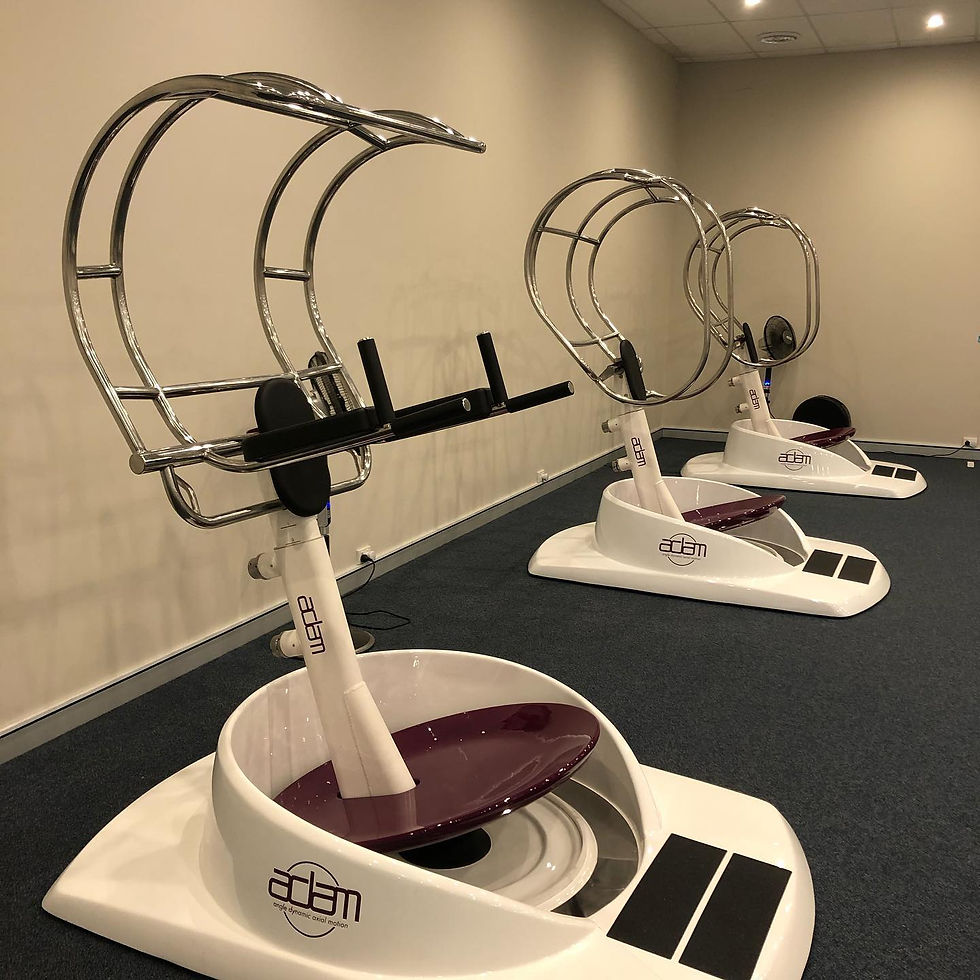
The ability to efficiently increase blood flow, tone and coordinate activity of multiple muscle groups throughout the body is undoubtedly playing a significant role in the recovery of strength, balance and mobility reported by patients treated at the Isodynamic’s Sydney pilot clinic.
However, the ability of REVIVER treatment to also activate dormant neural signalling pathways or promote the formation of new beneficial neural circuits in the brain and spinal cord (2), (3), a process known as “neuroplasticity”, may also contribute to the recovery of both physical and mental faculties reported by patients.
Through robustly stimulating coordinated activation of the vestibular “balance” system and multiple muscle groups throughout the body, REVIVER treatment has the potential to stimulate the nervous system by a process called “neuromodulation” that is known to drive neuroplasticity (4), (5).
Promoting beneficial neuroplasticity is widely accepted as a promising therapeutic approach for neurological disorders such as Parkinson’s disease (6), stroke (7), cerebral palsy (8), (9), and brain (4) and spinal cord injury (10) and may perhaps explain/contribute towards the recovery reported by patients with these disorders after the application of REVIVER treatment at the Sydney Clinic.
REVIVER, Gravity and Epigenetics
Epigenetics can be defined as changes in cells and organs of the body caused by environmental stimuli that can modify gene expression without directly altering the genetic code itself (11), (12).
Gravity has been shown to promote epigenetic adaptions in cells and organs of plants and animals (13). Scientific studies of the effects of long-term space travel have in large part driven research into the effects of gravity on the nervous system (14) and other tissues of the body (15). Notably, changes in gravity can alter growth of axons (nerve fibres) (16), a key element of neuroplasticity.
At Isodynamics we consider the REVIVER to be a device for generating beneficial “epigenetic” effects of gravity in humans.
Future laboratory and clinic-based research will determine the epigenetic cellular adaptions to the radial motion “off axis” gravity stimuli provided by the REVIVER motion on the nervous system and other organs of the body.
References:
1. Allum, J.H., F. Honegger, and H. Acuna, Differential control of leg and trunk muscle activity by vestibulo-spinal and proprioceptive signals during human balance corrections. Acta Otolaryngol, 1995. 115(2): p. 124-9.
2. Brown, A.R. and M. Martinez, From cortex to cord: motor circuit plasticity after spinal cord injury. Neural Regen Res, 2019. 14(12): p. 2054-2062.
3. Xerri, C., Plasticity of cortical maps: multiple triggers for adaptive reorganization following brain damage and spinal cord injury. Neuroscientist, 2012. 18(2): p. 133-48.
4. D'Arcy, R.C.N., et al., Portable neuromodulation induces neuroplasticity to re-activate motor function recovery from brain injury: a high-density MEG case study. J Neuroeng Rehabil, 2020. 17(1): p. 158.
5. Hamilton, R.H., Neuroplasticity in the language system: Reorganization in post-stroke aphasia and in neuromodulation interventions. Restor Neurol Neurosci, 2016. 34(4): p. 467-71.
6. Johansson, H., et al., Exercise-Induced Neuroplasticity in Parkinson's Disease: A Metasynthesis of the Literature. Neural Plast, 2020. 2020: p. 8961493.
7. Xing, Y. and Y. Bai, A Review of Exercise-Induced Neuroplasticity in Ischemic Stroke: Pathology and Mechanisms. Mol Neurobiol, 2020. 57(10): p. 4218-4231.
8. Reid, L.B., et al., Measuring neuroplasticity associated with cerebral palsy rehabilitation: An MRI based power analysis. Int J Dev Neurosci, 2017. 58: p. 17-25.
9. Reid, L.B., S.E. Rose, and R.N. Boyd, Rehabilitation and neuroplasticity in children with unilateral cerebral palsy. Nat Rev Neurol, 2015. 11(7): p. 390-400.
10. Loy, K. and F.M. Bareyre, Rehabilitation following spinal cord injury: how animal models can help our understanding of exercise-induced neuroplasticity. Neural Regen Res, 2019. 14(3): p. 405-412.
11. Berger, S.L., et al., An operational definition of epigenetics. Genes Dev, 2009. 23(7): p. 781-3.
12. Cavalli, G. and E. Heard, Advances in epigenetics link genetics to the environment and disease. Nature, 2019. 571(7766): p. 489-499.
13. Takahashi, K., et al., Gravity sensing in plant and animal cells. NPJ Microgravity, 2021. 7(1): p. 2.
14. Buoite Stella, A., et al., Neurophysiological adaptations to spaceflight and simulated microgravity. Clin Neurophysiol, 2021. 132(2): p. 498-504.
15. Shiba, N., et al., Electrically Stimulated Antagonist Muscle Contraction Increased Muscle Mass and Bone Mineral Density of One Astronaut - Initial Verification on the International Space Station. PLoS One, 2015. 10(8): p. e0134736.
16. Kohn, F.P.M. and R. Ritzmann, Gravity and neuronal adaptation, in vitro and in vivo-from neuronal cells up to neuromuscular responses: a first model. Eur Biophys J, 2018. 47(2): p. 97-107.
Comments